Excitation features of surface acoustic waves by interdigital transducer in piezoelectric crystals
DOI:
https://doi.org/10.3103/S0735272721080033Keywords:
piezoelectric, surface acoustic wave, SAW, monocrystalAbstract
The mathematical models of a long electrode with finite cross-section dimensions, an electrode pair and an interdigital transducer in the excitation mode of surface acoustic waves in Z-sections of piezoelectric crystals of the 6mm crystallographic class are proposed in this article. The accounting of finite dimensions of the electrodes cross-section makes corrections in the numerical values of the synchronism frequency and the level of radiated surface waves. The analytical expressions are obtained and the effect of the electrode cross-section dimensions in electrode pair on the synchronism frequency, the amplitude of the surface acoustic wave, and the module of wave characteristic of interdigital transducer are investigated. We prove that at zero electrode thickness, the maximum displacement level in surface acoustic wave is of 9.75%, and for the square electrode section is 37.25% less than the value obtained by the δ-sources method. At the same time, the synchronism frequency decreases by 9% in the first case. With the electrode thickness equal to the half-width, the real value of synchronism frequency is of 21.7%, and the surface wave amplitude is 33.1% less than in the case of the δ-sources method.
References
C. Caliendo, M. Hamidullah, “Guided acoustic wave sensors for liquid environments,” J. Phys. D Appl. Phys., vol. 52, no. 15, p. 153001, 2019, doi: https://doi.org/10.1088/1361-6463/aafd0b.
C. Caliendo, M. Hamidullah, “Pressure sensing with zero group velocity lamb modes in self-supported a-SiC/c-ZnO membranes,” J. Phys. D Appl. Phys., vol. 51, no. 38, p. 385102, 2018, doi: https://doi.org/10.1088/1361-6463/aad6f3.
A. Mujahid, F. L. Dickert, “Surface acoustic wave (SAW) for chemical sensing applications of recognition layers,” Sensors (Switzerlad), vol. 17, no. 12, p. 2716, 2017, doi: https://doi.org/10.3390/s17122716.
Y. Zhang, F. Yang, Z. Sun, Y.-T. Li, G.-J. Zhang, “A surface acoustic wave biosensor synergizing DNA-mediated in situ silver nanoparticle growth for a highly specific and signal-amplified nucleic acid assay,” Analyst, vol. 142, no. 18, pp. 3468–3476, 2017, doi: https://doi.org/10.1039/C7AN00988G.
A. Marcu, C. Viespe, “Surface acoustic wave sensors for hydrogen and deuterium detection,” Sensors, vol. 17, no. 6, p. 1417, 2017, doi: https://doi.org/10.3390/s17061417.
W. Xuan et al., “Fast response and high sensitivity ZnO/glass surface acoustic wave humidity sensors using graphene oxide sensing layer,” Sci. Reports, vol. 4, no. 1, p. 7206, 2015, doi: https://doi.org/10.1038/srep07206.
A. C. Poveda, D. D. Bühler, A. C. Sáez, P. V. Santos, M. M. de Lima, “Semiconductor optical waveguide devices modulated by surface acoustic waves,” J. Phys. D Appl. Phys., vol. 52, no. 25, p. 253001, 2019, doi: https://doi.org/10.1088/1361-6463/ab1464.
M. Weiß, H. J. Krenner, “Interfacing quantum emitters with propagating surface acoustic waves,” J. Phys. D Appl. Phys., vol. 51, no. 37, p. 373001, 2018, doi: https://doi.org/10.1088/1361-6463/aace3c.
A. V. Varlamov, V. V. Lebedev, P. M. Agruzov, I. V. Ilichev, L. V. Shamrai, A. V. Shamrai, “Acousto-optic frequency shift modulators with acoustic and optic waveguides on X-cut lithium niobate substrates,” J. Phys. Conf. Ser., vol. 132612011, 2019, doi: https://doi.org/10.1088/1742-6596/1326/1/012011.
E. D. S. Nysten, Y. H. Huo, H. Yu, G. F. Song, A. Rastelli, H. J. Krenner, “Multi-harmonic quantum dot optomechanics in fused LiNbO3–(Al)GaAs hybrids,” J. Phys. D Appl. Phys., vol. 50, no. 43, p. 43LT01, 2017, doi: https://doi.org/10.1088/1361-6463/aa861a.
R. Fandan, J. Pedrós, J. Schiefele, A. Boscá, J. Martínez, F. Calle, “Acoustically-driven surface and hyperbolic plasmon-phonon polaritons in graphene/h-BN heterostructures on piezoelectric substrates,” J. Phys. D Appl. Phys., vol. 51, no. 20, p. 204004, 2018, doi: https://doi.org/10.1088/1361-6463/aab8bd.
P. Delsing et al., “The 2019 surface acoustic waves roadmap,” J. Phys. D Appl. Phys., vol. 52, no. 35, p. 353001, 2019, doi: https://doi.org/10.1088/1361-6463/ab1b04.
J. H. Kuypers, A. P. Pisano, “Green’s function analysis of Lamb wave resonators,” in 2008 IEEE Ultrasonics Symposium, 2008, pp. 1548–1551, doi: https://doi.org/10.1109/ULTSYM.2008.0377.
V. K. Tewary, “Green’s-function method for modeling surface acoustic wave dispersion in anisotropic material systems and determination of material parameters,” Wave Motion, vol. 40, no. 4, pp. 399–412, 2004, doi: https://doi.org/10.1016/j.wavemoti.2004.02.007.
N. Nama, R. Barnkob, Z. Mao, C. J. Kähler, F. Costanzo, T. J. Huang, “Numerical study of acoustophoretic motion of particles in a PDMS microchannel driven by surface acoustic waves,” Lab a Chip, vol. 15, no. 12, pp. 2700–2709, 2015, doi: https://doi.org/10.1039/C5LC00231A.
T. Wang et al., “Surface acoustic waves (SAW)-based biosensing for quantification of cell growth in 2D and 3D cultures,” Sensors, vol. 15, no. 12, pp. 32045–32055, 2015, doi: https://doi.org/10.3390/s151229909.
S. Padilla, E. Tufekcioglu, R. Guldiken, “Simulation and verification of polydimethylsiloxane (PDMS) channels on acoustic microfluidic devices,” Microsyst. Technol., vol. 24, no. 8, pp. 3503–3512, 2018, doi: https://doi.org/10.1007/s00542-018-3760-2.
K. M. M. Kabir, G. I. Matthews, Y. M. Sabri, S. P. Russo, S. J. Ippolito, S. K. Bhargava, “Development and experimental verification of a finite element method for accurate analysis of a surface acoustic wave device,” Smart Mater. Struct., vol. 25, no. 335040, 2016, doi: https://doi.org/10.1088/0964-1726/25/3/035040.
T. Wang, R. Green, R. Guldiken, J. Wang, S. Mohapatra, S. S. Mohapatra, “Finite element analysis for surface acoustic wave device characteristic properties and sensitivity,” Sensors, vol. 19, no. 8, p. 1749, 2019, doi: https://doi.org/10.3390/s19081749.
K.-C. Park, J. R. Yoon, “Transmission line matrix modeling for analysis of surface acoustic wave hydrogen sensor,” Japanese J. Appl. Phys., vol. 50, no. 77HD06, 2011, doi: https://doi.org/10.1143/JJAP.50.07HD06.
T. Kojima, H. Obara, K. Shibayama, “Investigation of impulse response for an interdigital surface-acoustic-wave transducer,” Japanese J. Appl. Phys., vol. 29, no. S1, p. 125, 1990, doi: https://doi.org/10.7567/JJAPS.29S1.125.
T. Hoang, “SAW parameters analysis and equivalent circuit of SAW device,” in Acoustic Waves - From Microdevices to Helioseismology, InTech, 2011.
T. Kojima, K. Shibayama, “An analysis of an equivalent circuit model for an interdigital surface-acoustic-wave transducer,” Japanese J. Appl. Phys., vol. 27, no. S1, p. 163, 1988, doi: https://doi.org/10.7567/JJAPS.27S1.163.
I. V. Linchevskyi, “Excitation of surface acoustic waves in a Z-section of piezoelectric crystals by the electric field of a long electrode,” Int. J. Appl. Phys., vol. 6, no. 3, pp. 42–50, 2019, doi: https://doi.org/10.14445/23500301/IJAP-V6I3P108.
I. V. Linchevskyi, O. N. Petrischev, “Surface acoustic waves in Z-sections of piezoelectric monocrystals of hexagonal syngony,” Radioelectron. Commun. Syst., vol. 63, no. 3, pp. 156–170, 2020, doi: https://doi.org/10.3103/S0735272720030048.
D. Morgan, Surface Acoustic Wave Filters. Academic Press, 2007, uri: https://www.elsevier.com/books/surface-acoustic-wave-filters/morgan/978-0-12-372537-0.
L. Wang, H. Wang, “Analysis of propagation characteristics of AlN/diamond/Si layered SAW resonator,” Microsyst. Technol., vol. 26, no. 4, pp. 1273–1283, 2020, doi: https://doi.org/10.1007/s00542-019-04658-y.
T. J. Matula, P. L. Marston, “Electromagnetic acoustic wave transducer for the generation of acoustic evanescent waves on membranes and optical and capacitor wave‐number selective detectors,” J. Acoust. Soc. Am., vol. 93, no. 4, pp. 2221–2227, 1993, doi: https://doi.org/10.1121/1.406683.
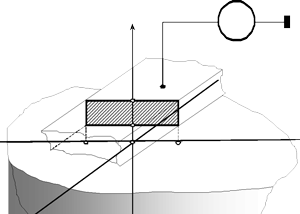