Theoretical estimates of vacuum conductivity of nonlinear channel of short-focus electron beam transport
DOI:
https://doi.org/10.3103/S0735272724010023Keywords:
electron beam transportation, nonlinear transport channel, vacuum conductivity, electron beam settling, current losses, high-voltage glow discharge, HVGD, electron gun, optimization of transport channel geometryAbstract
The paper presents analytical relationships obtained for calculating the vacuum conductivity of a nonlinear electron beam transport channel, the geometry of which is described by the power law dependence of the channel radius on the longitudinal coordinate. The dependences of the length of the transport channel on the pressure in the process chamber and also on the inlet and outlet radii of the channel are derived and analyzed. The obtained theoretical results are of practical importance for designing sputtering and welding electron beam equipment based on high-voltage glow discharge electron guns. The results of calculated data have been verified experimentally for a cylindrical transportation channel with an input diaphragm. To harmonize the theoretical and experimental data, a correction factor was introduced into the calculation formula to determine the length of the channel, and the value of this factor was adjusted based on the results of the experimental studies. The resulting relative difference between the calculated and experimental data does not exceed 15%. The calculated results obtained in this study can be used to optimize the geometry of the transport channel to achieve minimal losses of electron beam current in the channel.
References
S. Schiller, U. Heisig, S. Panzer, Electron Beam Technology. New-York: John Wiley & Sons, 1982.
M. Szilagyi, Electron and Ion Optics. Springer, 2011.
S. V. Denbnovetsky, I. V. Melnyk, V. G. Melnyk, B. A. Tugai, S. B. Tuhai, “High voltage glow discharge electron guns and its advanced application examples in electronic industry,” in 2016 International Conference Radio Electronics & Info Communications (UkrMiCo), 2016, pp. 1–4, doi: https://doi.org/10.1109/UkrMiCo.2016.7739615.
S. J. R. Humphries, Charged Particle Beams. Courier Corporation, 2013.
T. O. Prikhna et al., “Electron-beam and plasma oxidation-resistant and thermal-barrier coatings deposited on turbine blades using cast and powder Ni(Co)CrALY(Si) alloys I. Fundamentals of the production technology, structure, and phase composition of cast NiCrAlY alloys,” Powder Metall. Met. Ceram., vol. 61, no. 1–2, pp. 70–76, 2022, doi: https://doi.org/10.1007/s11106-022-00296-8.
T. O. Prikhna et al., “Electron-beam and plasma oxidation-resistant and thermal-barrier coatings deposited on turbine blades using cast and powder Ni(Co)CrAlY(Si) alloys produced by electron-beam melting II. Structure and chemical and phase composition of cast CoCrAlY alloys,” Powder Metall. Met. Ceram., vol. 61, no. 3–4, pp. 230–237, 2022, doi: https://doi.org/10.1007/s11106-022-00310-z.
I. M. Grechanyuk et al., “Electron-beam and plasma oxidation-resistant and thermal-barrier coatings deposited on turbine blades using cast and powder Ni(Co)CrAlY(Si) alloys produced by electron beam melting IV. Chemical and phase composition and structure of cocralysi powder alloy,” Powder Metall. Met. Ceram., vol. 61, no. 7–8, pp. 459–464, 2022, doi: https://doi.org/10.1007/s11106-023-00333-0.
M. I. Grechanyuk et al., “Electron-beam and plasma oxidation-resistant and thermal-barrier coatings deposited on turbine blades using cast and powder Ni (Co)CrAlY (Si) aslloys produced by electron beam melting III. Formation, structure, and chemical and phase composition of therma,” Powder Metall. Met. Ceram., vol. 61, no. 5–6, pp. 328–336, 2022, doi: https://doi.org/10.1007/s11106-022-00320-x.
V. G. Grechanyuk et al., “Сopper and molybdenum-based nanocrystalline materials,” Metallofiz. i Noveishie Tekhnologii, vol. 44, no. 7, pp. 927–942, 2022, doi: https://doi.org/10.15407/mfint.44.07.0927.
M. I. Grechanyuk et al., “Massive microporous composites condensed from the vapour phase,” Nanosistemi, Nanomater. Nanotehnologii, vol. 20, no. 4, pp. 883–894, 2022, uri: https://www.imp.kiev.ua/nanosys/media/pdf/2022/4/nano_vol20_iss4_p0883p0894_2022.pdf.
M. I. Grechanyuk, V. G. Grechanyuk, A. M. Manulyk, I. M. Grechanyuk, A. V. Kozyrev, V. I. Gots, “Massive dispersion-strengthened composition materials with metal matrix condensed from the vapour phase,” Nanosistemi, Nanomater. Nanotehnologii, vol. 20, no. 3, pp. 683–692, 2022, uri: https://www.imp.kiev.ua/nanosys/media/pdf/2022/3/nano_vol20_iss3_p0683p0692_2022.pdf.
N. I. Grechanyuk, V. P. Konoval, V. G. Grechanyuk, G. A. Bagliuk, D. V. Myroniuk, “Properties of Cu–Mo materials produced by physical vapor deposition for electrical contacts,” Powder Metall. Met. Ceram., vol. 60, no. 3–4, pp. 183–190, 2021, doi: https://doi.org/10.1007/s11106-021-00226-0.
N. I. Grechanyuk, V. G. Grechanyuk, “Precipitation-strengthened and microlayered bulk copper- and molybdenum-based nanocrystalline materials produced by high-speed electron-beam evaporation–condensation in vacuum: structure and phase composition,” Powder Metall. Met. Ceram., vol. 56, no. 11–12, pp. 633–646, 2018, doi: https://doi.org/10.1007/s11106-018-9938-4.
N. I. Grechanyuk et al., “Laboratory electron-beam multipurpose installation L-2 for producing alloys, composites, coatings, and powders,” Powder Metall. Met. Ceram., vol. 56, no. 1–2, pp. 113–121, 2017, doi: https://doi.org/10.1007/s11106-017-9878-4.
A. Zakharov, S. Rozenko, S. Litvintsev, M. Ilchenko, “Trisection bandpass filter with mixed cross-coupling and different paths for signal propagation,” IEEE Microw. Wirel. Components Lett., vol. 30, no. 1, pp. 12–15, 2020, doi: https://doi.org/10.1109/LMWC.2019.2957207.
A. Zakharov, S. Rozenko, M. Ilchenko, “Varactor-tuned microstrip bandpass filter with loop hairpin and combline resonators,” IEEE Trans. Circuits Syst. II Express Briefs, vol. 66, no. 6, pp. 953–957, 2019, doi: https://doi.org/10.1109/TCSII.2018.2873227.
A. A. Druzhinin, I. P. Ostrovskii, Y. N. Khoverko, N. S. Liakh-Kaguy, A. M. Vuytsyk, “Low temperature characteristics of germanium whiskers,” Funct. Mater., vol. 21, no. 2, pp. 130–136, 2014, doi: https://doi.org/10.15407/fm21.02.130.
A. Druzhinin, I. Bolshakova, I. Ostrovskii, Y. Khoverko, N. Liakh-Kaguy, “Low temperature magnetoresistance of InSb whiskers,” Mater. Sci. Semicond. Process., vol. 40, pp. 550–555, 2015, doi: https://doi.org/10.1016/j.mssp.2015.07.030.
A. N. Kalinuk, A. Y. Derecha, V. V. Telin, A. F. Kolyada, V. I. Kostenko, N. M. Ivanov, “Peculiarities of production of VT1-0 and GRADE 2 cast strip blanks from low-grade spongy titanium,” Adv. Electrometallyrgy, no. 3, pp. 20–26, 2018.
T. Kemmotsu, T. Nagai, M. Maeda, “Removal rate of phosphorus from molten silicon,” High Temp. Mater. Process., vol. 30, no. 1–2, pp. 17–22, 2011, doi: https://doi.org/10.1515/htmp.2011.002.
M. Armstrong, H. Mehrabi, N. Naveed, “An overview of modern metal additive manufacturing technology,” J. Manuf. Process., vol. 84, pp. 1001–1029, 2022, doi: https://doi.org/10.1016/j.jmapro.2022.10.060.
W. E. Frazier, “Metal additive manufacturing: a review,” J. Mater. Eng. Perform., vol. 23, no. 6, pp. 1917–1928, 2014, doi: https://doi.org/10.1007/s11665-014-0958-z.
Additive Manufacturing for the Aerospace Industry. Elsevier, 2019, doi: https://doi.org/10.1016/C2017-0-00712-7.
R. Brooke, “Additive manufacturing can lower aircraft building and operating costs,” TCT mag, 2013.
D. Kovalchuk et al., “Microstructure and properties of Ti-6Al-4V articles 3D-printed with co-axial electron beam and wire technology,” J. Mater. Eng. Perform., vol. 30, no. 7, pp. 5307–5322, 2021, doi: https://doi.org/10.1007/s11665-021-05770-9.
I. Melnyk, S. Tuhai, M. Surzhykov, I. Shved, V. Melnyk, D. Kovalchuk, “Analytical estimation of the deep of seam penetration for the electron-beam welding technologies with application of glow discharge electron guns,” in 2022 IEEE 41st International Conference on Electronics and Nanotechnology (ELNANO), 2022, pp. 1–5, doi: https://doi.org/10.1109/ELNANO54667.2022.9927071.
I. V. Melnyk, “Simulation of geometry of high voltage glow discharge electrodes’ systems, formed profile electron beams,” in Proc. SPIE 6278, Seventh Seminar on Problems of Theoretical and Applied Electron and Ion Optics, 2006, pp. 627809-627809–13, doi: https://doi.org/10.1117/12.693202.
I. V. Melnyk, A. V. Pochynok, “Modeling of electron sources for high voltage glow discharge forming profiled electron beams,” Radioelectron. Commun. Syst., vol. 62, no. 6, pp. 251–261, 2019, doi: https://doi.org/10.3103/S0735272719060013.
I. V. Melnyk, V. G. Melnyk, B. A. Tugai, S. B. Tuhai, N. I. Mieshkova, A. V. Pochynok, “Simplyfied universal analytical model for defining of plasma boundary position in the glow discharge electron guns for forming conic hollow electron beam,” in 2019 IEEE 39th International Conference on Electronics and Nanotechnology (ELNANO), 2019, pp. 548–552, doi: https://doi.org/10.1109/ELNANO.2019.8783454.
S. V. Denbnovetsky, V. I. Melnik, I. V. Melnik, K. A. Tugay, “Investigation of forming of electron beam in glow discharge electron guns with additional electrode,” in Proceedings ISDEIV. 18th International Symposium on Discharges and Electrical Insulation in Vacuum (Cat. No.98CH36073), 1998, vol. 2, pp. 637–640, doi: https://doi.org/10.1109/DEIV.1998.738827.
S. V. Denbnovetskiy, V. G. Melnyk, I. V. Melnyk, B. A. Tugay, S. B. Tugay, “Investigation of electron-ion optics of pulse technological glow discharge electron guns,” in 2013 IEEE XXXIII International Scientific Conference Electronics and Nanotechnology (ELNANO), 2013, pp. 420–424, doi: https://doi.org/10.1109/ELNANO.2013.6552052.
P. Hawkes, E. Kasper, Principles of Electron Optics. Elsevier, 2018, doi: https://doi.org/10.1016/C2015-0-06653-9.
P. Grivet, Electron Optics. Elsevier, 1972, doi: https://doi.org/10.1016/C2013-0-02400-0.
J. Orloff, Handbook of Charged Particle Optics. CRC Press, 2009, uri: https://www.routledge.com/Handbook-of-Charged-Particle-Optics/Orloff/p/book/9781420045543.
A. B. El-Kareh, J. C. J. El-Kareh, Electron Beams, Lenses, and Optics. Elsevier, 1970, doi: https://doi.org/10.1016/B978-0-12-238001-3.X5001-3.
J. T. L. Thong, Ed., Electron Beam Testing Technology. Boston, MA: Springer US, 1993, doi: https://doi.org/10.1007/978-1-4899-1522-1.
B. M. Smirnov, Theory of Gas Discharge Plasma, vol. 84. Cham: Springer International Publishing, 2015, doi: https://doi.org/10.1007/978-3-319-11065-3.
M. A. Lieberman, A. J. Lichtenberg, Principles of Plasma Discharges and Materials Processing. Wiley, 2005, doi: https://doi.org/10.1002/0471724254.
Y. P. Raizer, Gas Discharge Physics. Berlin, Heidelberg: Springer Berlin Heidelberg, 1991, doi: https://doi.org/10.1007/978-3-642-61247-3.
I. V. Melnyk, “Numerical simulation of distribution of electric field and particle trajectories in electron sources based on high-voltage glow discharge,” Radioelectron. Commun. Syst., vol. 48, no. 6, pp. 41–48, 2005, doi: https://doi.org/10.3103/S0735272705060087.
I. Melnyk, S. Tuhai, M. Surzhykov, I. Shved, V. Melnyk, D. Kovalchuk, “Different approaches for analytic and numerical estimation of operation temperature of cooled cathode surface in high voltage glow discharge electron guns,” in Progress in Advanced Information and Communication Technology and Systems. MCiT 2021. Lecture Notes in Networks and Systems, Cham: Springer, 2023, pp. 575–595.
I. Melnyk, S. Tuhai, M. Surzhykov, V. Kyryk, I. Shved, “Estimation of operation temperature of cooled cathode surface in technological high voltage glow discharge electron guns : invited paper,” in 2021 IEEE International Conference on Information and Telecommunication Technologies and Radio Electronics (UkrMiCo), 2021, pp. 258–262, doi: https://doi.org/10.1109/UkrMiCo52950.2021.9716698.
S. V. Denbnovetsky, I. V. Melnyk, V. G. Melnyk, B. A. Tugai, S. B. Tuhai, “Simulation of dependences of discharge current of high voltage glow discharge electron guns from parameters of electromagnetic valve,” in 2017 IEEE 37th International Conference on Electronics and Nanotechnology (ELNANO), 2017, pp. 369–373, doi: https://doi.org/10.1109/ELNANO.2017.7939781.
I. V. Melnyk, V. G. Melnyk, B. A. Tugai, S. B. Tuhai, “Investigation of complex control system for high voltage glow discharge electron sources,” in 2017 International Conference on Information and Telecommunication Technologies and Radio Electronics (UkrMiCo), 2017, pp. 1–5, doi: https://doi.org/10.1109/UkrMiCo.2017.8095394.
I. V. Melnyk, “Simulation of energetic efficiency of triode high voltage glow discharge electron sources with account of temperature of electrons and its mobility in anode plasma,” Radioelectron. Commun. Syst., vol. 60, no. 7, pp. 319–329, 2017, doi: https://doi.org/10.3103/S0735272717070056.
I. Melnyk, S. Tuhai, M. Surzhykov, I. Shved, “Discrete vehicle automation algorithm based on the theory of finite state machine,” in Emerging Networking in the Digital Transformation Age. TCSET 2022. Lecture Notes in Electrical Engineering, Cham: Springer, 2023, pp. 231–245.
G. Lewin, Fundamentals of Vacuum Science and Technology. McGraw-Hill, 1965, uri: https://www.amazon.com/Fundamentals-Vacuum-Science-Technology-Gerhard/dp/B0000CMJUA.
J. M. Lafferty, Foundations of Vacuum Science and Technology. Wiley, 1998, uri: https://www.wiley.com/en-us/Foundations+of+Vacuum+Science+and+Technology-p-9780471175933.
M. H. Hablanian, High-Vacuum Technology. New York: Routledge, 2017, doi: https://doi.org/10.1201/9780203751923.
K. Jousten, Handbook of Vacuum Technology. Wiley, 2016, doi: https://doi.org/10.1002/9783527688265.
D. M. Hata, E. V. Brewer, N. J. Louwagie, Introduction to Vacuum Technology. Milne Open Textbooks, 2023, uri: https://open.umn.edu/opentextbooks/textbooks/introduction-to-vacuum-technology.
D. J. Hucknall, Vacuum Technology and Applications. Elsevier, 1991, doi: https://doi.org/10.1016/C2013-0-01220-0.
P. K. Naik, Vacuum: Science, Technology and Applications. CRC Press, 2018, uri: https://www.routledge.com/Vacuum-Science-Technology-and-Applications/Naik/p/book/9780367572068.
I. N. Bronshtein, K. A. Semendyayev, G. Musiol, H. Mühlig, Handbook of Mathematics. Berlin, Heidelberg: Springer Berlin Heidelberg, 2015, doi: https://doi.org/10.1007/978-3-662-46221-8.
M. Abramowitz, I. A. Stegun, Handbook of Mathematical Functions with Formulas, Graphs and Mathematical Tables. Gaithersburg: National Bureau of Standards, 1964.
I. Melnyk, A. Luntovskyy, “Estimation of energy efficiency and quality of service in cloud realizations of parallel computing algorithms for IBN,” in Future Intent-Based Networking. Lecture Notes in Electrical Engineering, Vol 831, Cham: Springer, 2022, pp. 339–379.
K. D. Mathews, J. H. Fink, Numerical Methods Using MATLAB. Prentice Hall, 1998, uri: https://www.amazon.com/Numerical-Methods-Using-MATLAB-3rd/dp/0132700425/.
G. M. Phillips, Interpolation and Approximation by Polynomials. New York, NY: Springer New York, 2003, doi: https://doi.org/10.1007/b97417.
N. R. Draper, H. Smith, Applied Regression Analysis. Wiley, 1998, doi: https://doi.org/10.1002/9781118625590.
C. Mohan, K. Deep, Optimization Techniques. New Academic Science, 2009, uri: https://www.amazon.com/Optimization-Techniques-C-Mohan/dp/1906574219.
M. K. Jain, Numerical Methods for Scientific & Engineering Computation. New Age International Pvt. Ltd., 2010.
S. Chapra, R. Canale, Numerical Methods for Engineers. McGraw Hill, 2021, uri: https://www.mheducation.com/highered/product/Numerical-Methods-for-Engineers-Chapra.html.
I. V. Melnyk, S. B. Tuhai, A. V. Pochynok, “Calculation of focal paramters of electron beam formed in soft vacuum at the plane which sloped to beam axis,” in 2019 International Conference on Information and Telecommunication Technologies and Radio Electronics (UkrMiCo), 2019, pp. 1–5, doi: https://doi.org/10.1109/UkrMiCo47782.2019.9165328.
I. V. Melnyk, A. V. Pochynok, “Investigation of the class of algebraical functions for interpolation of boundary trajectories of short-focus electron beams,” Syst. Res. Inf. Technol., no. 3, pp. 23–39, 2020, doi: https://doi.org/10.20535/SRIT.2308-8893.2020.3.02.
I. Melnyk, A. Pochynok, “Basic algorithm for approximation of the boundary trajectory of short-focus electron beam using the root-polynomial functions of the fourth and fifth order,” Syst. Res. Inf. Technol., no. 3, pp. 127–148, 2023, doi: https://doi.org/10.20535/SRIT.2308-8893.2023.3.10.
I. Melnyk, S. Tuhai, M. Skrypka, T. Khyzhniak, A. Pochynok, “A new approach to interpolation and approximation of boundary trajectories of electron beams for realizing cloud computing using root-polynomial functions,” in Information and Communication Technologies and Sustainable Development. ICT&SD 2022. Lecture Notes in Networks and Systems, Vol 809, Cham: Springer, 2023, pp. 395–427.
I. Melnyk, S. Tuhai, M. Skrypka, A. Pochynok, D. Kovalchuk, “Approximation of the boundary trajectory of a short-focus electron beam using third-order root-polynomial functions and recurrent matrixes approach,” in 2023 International Conference on Information and Digital Technologies (IDT), 2023, pp. 133–138, doi: https://doi.org/10.1109/IDT59031.2023.10194399.
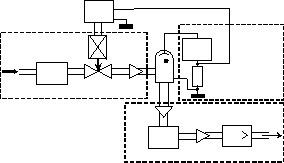