Theoretical justification of application possibility of different order root-polynomial functions for interpolation and approximation of boundary trajectory of electron beam
DOI:
https://doi.org/10.3103/S0735272723020024Keywords:
electron guns, high-voltage glow discharge, transport of electron beams, space charge, compensation of space charge, residual gas, functional analysis, interpolation, approximation, root-polynomial functionsAbstract
In this paper on a basis of functional analysis methods we justified theoretically the possibility of different orders root-polynomial functions application for interpolation and approximation of the boundary trajectory of an electron beam in case of its propagation in ionized gas with compensation of the space charge of the beam electrons. It is shown, that the root-polynomial functions satisfy to the second-order differential equation, describing the boundary trajectory of the beam electrons under such physical conditions. The results of interpolation and approximation of the boundary trajectory of the electron beam by root-polynomial functions from the second to the fifth order under the following physical conditions are presented. The interpolation results are compared with the corresponded results of the differential equation solution for the boundary trajectory of the electron beam using Runge-Kutta numerical method of the fourth order. These results are considered as reference ones for the interpolation task. To solve the approximation problem, in this paper an iterative algorithm based on the calculation of both values of the function and its derivatives at reference points is proposed. The approximation task is solved for a sample of numerical data obtained by experimental electron-beam equipment for real processes of current electron-beam technologies, which led to a rather large value of the experimental measurement error due to the effect of random factors associated with thermal treatment of products with electron beam. Test calculations show that the error of interpolation and approximation of numerical data, describing the boundary trajectory of electron beam in case of its propagation in ionized gas, does not exceed a few percent. The theoretical and practical results obtained in this paper are interesting for a wide range of specialists who are engaged in the physics of electron beams, the development of electron-beam technological equipment and implementation of current electron-beam technologies into industry.
References
I. Melnyk, S. Tyhai, A. Pochynok, “Universal complex model for estimation the beam current density of high voltage glow discharge electron guns,” in Lecture Notes in Networks and Systems, 2021, pp. 319–341.
S. Denbnovetsky et al., “Principles of operation of high voltage glow discharge electron guns and some possibilities of their technological application,” in Proceedings of SPIE, 2017, p. 104455R, doi: https://doi.org/10.1117/12.2280736.
S. V. Denbnovetsky, V. G. Melnyk, I. V. Melnyk, “High-voltage, glow-discharge electron sources and possibilities of its application in industry for realizing different technological operations,” IEEE Trans. Plasma Sci., vol. 31, no. 5, pp. 987–993, 2003, doi: https://doi.org/10.1109/TPS.2003.818444.
S. V. Denbnovetsky, V. I. Melnyk, I. V. Melnyk, B. A. Tugay, “Model of control of glow discharge electron gun current for microelectronics production applications,” in Sixth International Conference on Material Science and Material Properties for Infrared Optoelectronics, 2003, p. 64, doi: https://doi.org/10.1117/12.502174.
I. V. Melnyk, “Estimating of current rise time of glow discharge in triode electrode system in case of control pulsing,” Radioelectron. Commun. Syst., vol. 56, no. 12, pp. 592–599, 2013, doi: https://doi.org/10.3103/S0735272713120066.
I. Khytruk, A. Druzhinin, I. Ostrovskii, Y. Khoverko, N. Liakh-Kaguy, K. Rogacki, “Properties of doped GaSb whiskers at low temperatures,” Nanoscale Res. Lett., vol. 12, no. 1, p. 156, 2017, doi: https://doi.org/10.1186/s11671-017-1923-1.
A. Druzhinin, I. Bolshakova, I. Ostrovskii, Y. Khoverko, N. Liakh-Kaguy, “Low temperature magnetoresistance of InSb whiskers,” Mater. Sci. Semicond. Process., vol. 40, pp. 550–555, 2015, doi: https://doi.org/10.1016/j.mssp.2015.07.030.
A. Zakharov, S. Rozenko, S. Litvintsev, M. Ilchenko, “Trisection bandpass filter with mixed cross-coupling and different paths for signal propagation,” IEEE Microw. Wirel. Components Lett., vol. 30, no. 1, pp. 12–15, 2020, doi: https://doi.org/10.1109/LMWC.2019.2957207.
A. Zakharov, S. Litvintsev, M. Ilchenko, “Trisection bandpass filters with all mixed couplings,” IEEE Microw. Wirel. Components Lett., vol. 29, no. 9, pp. 592–594, 2019, doi: https://doi.org/10.1109/LMWC.2019.2929650.
A. Zakharov, S. Rozenko, M. Ilchenko, “Varactor-tuned microstrip bandpass filter with loop hairpin and combline resonators,” IEEE Trans. Circuits Syst. II Express Briefs, vol. 66, no. 6, pp. 953–957, 2019, doi: https://doi.org/10.1109/TCSII.2018.2873227.
A. Zakharov, S. Litvintsev, M. Ilchenko, “Transmission line tunable resonators with intersecting resonance regions,” IEEE Trans. Circuits Syst. II Express Briefs, vol. 67, no. 4, pp. 660–664, 2020, doi: https://doi.org/10.1109/TCSII.2019.2922429.
M. I. Grechanyuk, A. G. Melnyk, I. M. Grechanyuk, V. G. Melnyk, D. V. Kovalchuk, “Modern electron beam technologies and equipment for melting of metals and alloys, deposition of protective coatings, production of composites condensed from vapor phase and powders,” Electrotech. Electron., vol. 49, no. 5–6, pp. 115–121, 2014, uri: https://epluse.ceec.bg/modern-electron-beam-technologies-and-equipment-for-melting-of-metals-and-alloys-deposition-of-protective-coatings-production-of-composites-condensed-from-vapor-phase-and-powders/.
G. Mattausch et al., “Gas discharge electron sources – proven and novel tools for thin-film technologies,” Electrotech. Electron., vol. 49, no. 5–6, pp. 183–195, 2014.
V. Vassilieva, K. Vutova, D. V., “Recycling of alloy steel by electron beam melting,” Electrotech. Electron., vol. 47, no. 5–6, pp. 142–145, 2012.
H. Sasaki, Y. Kobashi, T. Nagai, M. Maeda, “Application of electron beam melting to the removal of phosphorus from silicon: toward production of solar-grade silicon by metallurgical processes,” Adv. Mater. Sci. Eng., vol. 2013, pp. 1–8, 2013, doi: https://doi.org/10.1155/2013/857196.
T. Kemmotsu, T. Nagai, M. Maeda, “Removal rate of phosphorus from molten silicon,” High Temp. Mater. Process., vol. 30, no. 1–2, pp. 17–22, 2011, doi: https://doi.org/10.1515/htmp.2011.002.
J. C. S. Pires, J. Otubo, A. F. B. Braga, P. R. Mei, “The purification of metallurgical grade silicon by electron beam melting,” J. Mater. Process. Technol., vol. 169, no. 1, pp. 16–20, 2005, doi: https://doi.org/10.1016/j.jmatprotec.2004.03.035.
D. Luo, N. Liu, Y. Lu, G. Zhang, T. Li, “Removal of impurities from metallurgical grade silicon by electron beam melting,” J. Semicond., vol. 32, no. 333003, 2011, doi: https://doi.org/10.1088/1674-4926/32/3/033003.
A. E. Davis, J. R. Kennedy, D. Strong, D. Kovalchuk, S. Porter, P. B. Prangnell, “Tailoring equiaxed β-grain structures in Ti-6Al-4V coaxial electron beam wire additive manufacturing,” Materialia, vol. 20, p. 101202, 2021, doi: https://doi.org/10.1016/j.mtla.2021.101202.
J. I. Etcheverry, N. Mingolo, J. J. Rocca, O. E. Martinez, “A simple model of a glow discharge electron beam for materials processing,” IEEE Trans. Plasma Sci., vol. 25, no. 3, pp. 427–432, 1997, doi: https://doi.org/10.1109/27.597256.
I. V. Melnyk, “Numerical simulation of distribution of electric field and particle trajectories in electron sources based on high-voltage glow discharge,” Radioelectron. Commun. Syst., vol. 48, no. 6, pp. 41–48, 2005, doi: https://doi.org/10.3103/S0735272705060087.
S. V. Denbnovetsky, J. Felba, V. I. Melnik, I. V. Melnik, “Model of beam formation in a glow discharge electron gun with a cold cathode,” Appl. Surf. Sci., vol. 111, pp. 288–294, 1997, doi: https://doi.org/10.1016/S0169-4332(96)00761-1.
J. D. Lawson, The Physics of Charged-Particle Beams. Oxford: Clarendon Press, 1977.
M. Reiser, Theory and Design of Charged Particle Beams. Wiley, 2008, uri: https://www.wiley.com/en-us/Theory+and+Design+of+Charged+Particle+Beams-p-9783527617630.
M. Szilagyi, Electron and Ion Optics. Springer, 2011.
S. Humphries, Charged Particle Beams. New York: Wiley-Interscience, 1990, uri: https://library.uoh.edu.iq/admin/ebooks/76728-charged-particle-beams---s.-humphries.pdf.
B. M. Smirnov, Theory of Gas Discharge Plasma. Springer, 2015, uri: https://www.amazon.com/Theory-Discharge-Springer-Optical-Physics/dp/3319110640.
M. A. Lieberman, A. J. Lichtenberg, Principles of Plasma Discharges and Materials Processing. Wiley, 2005, uri: https://www.wiley.com/en-us/Principles+of+Plasma+Discharges+and+Materials+Processing%2C+2nd+Edition-p-9780471720010.
Y. P. Raizer, Gas Discharge Physics. Berlin: Springer-Verlag, 1991.
I. V. Melnyk, “Simulation of energetic efficiency of triode high voltage glow discharge electron sources with account of temperature of electrons and its mobility in anode plasma,” Radioelectron. Commun. Syst., vol. 60, no. 7, pp. 319–329, 2017, doi: https://doi.org/10.3103/S0735272717070056.
I. Melnyk, S. Tuhai, A. Pochynok, “Interpolation of the boundary trajectories of electron beams by the roots from polynomic functions of corresponded order,” in 2020 IEEE 40th International Conference on Electronics and Nanotechnology (ELNANO), 2020, pp. 28–33, doi: https://doi.org/10.1109/ELNANO50318.2020.9088786.
I. V. Melnyk, S. B. Tuhai, A. V. Pochynok, “Interpolation functions for describing the boundary trajectories of electron beams propagated in ionised gas,” in 2020 IEEE 15th International Conference on Advanced Trends in Radioelectronics, Telecommunications and Computer Engineering (TCSET), 2020, pp. 79–83, doi: https://doi.org/10.1109/TCSET49122.2020.235395.
I. V. Melnyk, S. B. Tuhai, A. V. Pochynok, “Calculation of focal paramters of electron beam formed in soft vacuum at the plane which sloped to beam axis,” in 2019 International Conference on Information and Telecommunication Technologies and Radio Electronics (UkrMiCo), 2019, pp. 1–5, doi: https://doi.org/10.1109/UkrMiCo47782.2019.9165328.
I. V. Melnyk, A. V. Pochynok, “Investigation of the class of algebraical functions for interpolation of boundary trajectories of short-focus electron beams,” Syst. Res. Inf. Technol., no. 3, pp. 23–39, 2020, doi: https://doi.org/10.20535/SRIT.2308-8993.2020.3.02.
A. F. Tseluyko, V. T. Lazurik, D. L. Ryabchikov, V. I. Maslov, I. N. Sereda, “Experimental study of radiation in the wavelength range 12.2–15.8 nm from a pulsed high-current plasma diode,” Plasma Phys. Reports, vol. 34, no. 11, pp. 963–968, 2008, doi: https://doi.org/10.1134/S1063780X0811010X.
V. G. Rudychev, V. T. Lazurik, Y. V. Rudychev, “Influence of the electron beams incidence angles on the depth-dose distribution of the irradiated object,” Radiat. Phys. Chem., vol. 186, p. 109527, 2021, doi: https://doi.org/10.1016/j.radphyschem.2021.109527.
V. M. Lazurik, V. T. Lazurik, G. Popov, Z. Zimek, “Two-parametric model of electron beam in computational dosimetry for radiation processing,” Radiat. Phys. Chem., vol. 124, pp. 230–234, 2016, doi: https://doi.org/10.1016/j.radphyschem.2015.12.004.
K. D. Mathews, J. H. Fink, Numerical Methods Using MATLAB. Prentice Hall, 1998, uri: https://www.amazon.com/Numerical-Methods-Using-MATLAB-3rd/dp/0132700425/.
I. N. Bronstein, K. A. Semendyaev, Handbook of Mathematics for Engineers and University Students, [in Russian]. Moscow: Nauka, Fizmatlit, 1986.
J. F. Epperson, An Introduction to Numerical Methods and Analysis. Wiley-Interscience, 2007.
E. Wentzel, L. Ovcharov, Applied Problems of Probability Theory. Moscow: Mir Publishers, 1986, uri: https://mirtitles.org/2022/06/03/applied-problems-in-probability-theory-wentzel-ovcharov/.
N. R. Draper, H. Smith, Applied Regression Analysis. Wiley, 1998, uri: https://www.wiley.com/en-us/Applied+Regression+Analysis,+3rd+Edition-p-9780471170822.
J. A. Gubner, Probability and Random Processes for Electrical and Computer Engineers. Cambridge, UK: Cambridge University Press, 2006.
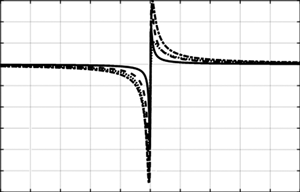