Charge transfer in polar crystals
DOI:
https://doi.org/10.3103/S0735272722120032Abstract
In some noncentrosymmetric crystals, their internal polarity can significantly influence the electric charge transfer. Sometimes, mixed ion-covalent bonds that are intrinsic to polar crystals are extremely delicately balanced, therefore, external stimuli (temperature or electric field) lead to a large change of the specific electrical resistance. In this case, even a small violation in the structure of materials stipulates a significant influence on electrical resistance, namely, a strong temperature dependence of the specific resistance of critistors and posistors and also field dependence of the specific resistance in varistors and controlled switching elements. A high sensitivity of specific resistance to peculiarities of the external environment that is stipulated by the imbalance of the internal polarity of active material is used in sensors. The analysis of the influence on electric charge transfer in polar structures can be crucial for the improvement of appropriate materials.
References
C. Kittel, Introduction to Solid State Physics. John Wiley & Sons, Inc., 2005.
M. E. Lines, A. M. Glass, Principles and Applications of Ferroelectrics and Related Materials. Oxford University Press, 2001, doi: https://doi.org/10.1093/acprof:oso/9780198507789.001.0001.
J. C. Burfoot, G. W. Taylor, Polar Dielectrics and Their Applications. University of California Press, 1979, doi: https://doi.org/10.1525/9780520315334.
Y. M. Poplavko, D. D. Tatarchuk, Y. V. Didenko, D. V. Chypegin, “Blurred relaxation spectra in dielectric materials,” Radioelectron. Commun. Syst., vol. 65, no. 5, pp. 221–234, 2022, doi: https://doi.org/10.3103/S0735272722050016.
R. E. Newnham, Properties of Materials: Anisotropy, Symmetry, Structure. Oxford: Oxford University Press, 2004, uri: https://global.oup.com/academic/product/properties-of-materials-9780198520764.
L. Levinson, H. R. Philipp, “Zinc oxide varistors - A review,” Am. Ceram. Soc. Bull., vol. 65, no. 4, pp. 639–646, 1986.
M. Inada, “Crystal phases of nonohmic zinc oxide ceramics,” Japanese J. Appl. Phys., vol. 17, no. 1, pp. 1–10, 1978, doi: https://doi.org/10.1143/JJAP.17.1.
Y.-B. Hahn, “Zinc oxide nanostructures and their applications,” Korean J. Chem. Eng., vol. 28, no. 9, pp. 1797–1813, 2011, doi: https://doi.org/10.1007/s11814-011-0213-3.
M. L. Kahn, M. Monge, V. Collière, F. Senocq, A. Maisonnat, B. Chaudret, “Size- and shape-control of crystalline zinc oxide nanoparticles: a new organometallic synthetic method,” Adv. Funct. Mater., vol. 15, no. 3, pp. 458–468, 2005, doi: https://doi.org/10.1002/adfm.200400113.
J. Fontcuberta, B. Martínez, A. Seffar, S. Piñol, J. L. García-Muñoz, X. Obradors, “Colossal magnetoresistance of ferromagnetic manganites: structural tuning and mechanisms,” Phys. Rev. Lett., vol. 76, no. 7, pp. 1122–1125, 1996, doi: https://doi.org/10.1103/PhysRevLett.76.1122.
A. P. Ramirez, “Colossal magnetoresistance,” J. Phys. Condens. Matter, vol. 9, no. 39, pp. 8171–8199, 1997, doi: https://doi.org/10.1088/0953-8984/9/39/005.
M. Uehara, S. Mori, C. H. Chen, S.-W. Cheong, “Percolative phase separation underlies colossal magnetoresistance in mixed-valent manganites,” Nature, vol. 399, no. 6736, pp. 560–563, 1999, doi: https://doi.org/10.1038/21142.
Y. M. Poplavko, Y. I. Yakymenko, “Large parameters and giant effects in electronic materials,” Radioelectron. Commun. Syst., vol. 63, no. 6, pp. 289–298, 2020, doi: https://doi.org/10.3103/S0735272720060023.
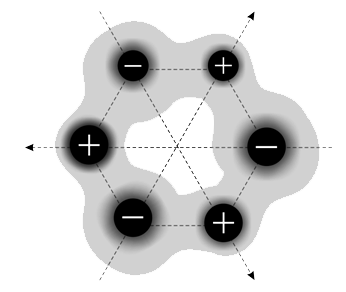