Design and analysis of AMC reflector integrated flexible and compact vehicular antenna for communication application
DOI:
https://doi.org/10.3103/S0735272722090059Keywords:
SRR, AMC reflectorAbstract
In this paper, a flexible, compact, and semi-transparent monopole antenna integrated with a 2×2 AMC reflector is presented for vehicular communication applications. F-shaped and inverted L-shaped stubs are connected to the radiator symmetrically with slots in the partial ground for making the antenna operate at a 5.85 GHz band. The antenna is designed using a flexible polyimide substrate (εr = 3.5, tanδ = 0.025) with dimensions of 14×14×0.5 mm3. An AMC reflector unit cell is designed using PDMS (Polydimethylsiloxane) substrate (εr = 2.66, tanδ = 0.023) with dimensions of 8×8×1 mm3. The AMC cell structure is designed with a split ring resonator (SRR) and rectangular ring with full ground resonating at 5.85 GHz. The 2×2 AMC reflector surface is integrated with a separation 9 mm to the designed antenna that provides the gain increment from 1.73 dBi to 4.56 dBi. The reflection coefficient S11 bandwidth of the AMC-backed antenna is 0.52 GHz, while the standalone antenna’s bandwidth is 0.44 GHz. The flexibility, compactness, and achieved radiation metrics project the proposed antenna structure a suitable choice for vehicular communication applications.
References
S. K. Bhoi, P. M. Khilar, “Vehicular communication: a survey,” IET Networks, vol. 3, no. 3, pp. 204–217, 2014, doi: https://doi.org/10.1049/iet-net.2013.0065.
L. Zhao et al., “Vehicular communications: Standardization and open issues,” IEEE Commun. Stand. Mag., vol. 2, no. 4, pp. 74–80, 2018, doi: https://doi.org/10.1109/MCOMSTD.2018.1800027.
M. Fallahpour, R. Zoughi, “Antenna miniaturization techniques: A review of topology- and material-based methods,” IEEE Antennas Propag. Mag., vol. 60, no. 1, pp. 38–50, 2018, doi: https://doi.org/10.1109/MAP.2017.2774138.
M. El Gharbi, R. Fernández-García, S. Ahyoud, I. Gil, “A review of flexible wearable antenna sensors: Design, fabrication methods, and applications,” Materials, vol. 13, no. 17, p. 3781, 2020, doi: https://doi.org/10.3390/ma13173781.
J. P. Gianvittorio, Y. Rahmat-Samii, “Fractal antennas: A novel antenna miniaturization technique, and applications,” IEEE Antennas Propag. Mag., vol. 44, no. 1, pp. 20–36, 2002, doi: https://doi.org/10.1109/74.997888.
B. T. P. Madhav, T. Anilkumar, S. K. Kotamraju, “Transparent and conformal wheel-shaped fractal antenna for vehicular communication applications,” AEU - Int. J. Electron. Commun., vol. 91, pp. 1–10, 2018, doi: https://doi.org/10.1016/j.aeue.2018.04.028.
C. A. Balanis, Antenna Theory: Analysis and Design. New Jersey: Wiley, 2016, uri: https://www.wiley.com/en-us/Antenna+Theory%3A+Analysis+and+Design%2C+4th+Edition-p-9781118642061.
R. Dhara, S. K. Jana, M. Mitra, “Tri-band circularly polarized monopole antenna for wireless communication application,” Radioelectron. Commun. Syst., vol. 63, no. 4, pp. 213–222, 2020, doi: https://doi.org/10.3103/S0735272720040044.
S. Lee, M. Choo, S. Jung, W. Hong, “Optically transparent nano-patterned antennas: A review and future directions,” Appl. Sci., vol. 8, no. 6, p. 901, 2018, doi: https://doi.org/10.3390/app8060901.
R. Dewan et al., “Artificial magnetic conductor for various antenna applications: An overview,” Int. J. RF Microw. Comput. Eng., vol. 27, no. 6, p. e21105, 2017, doi: https://doi.org/10.1002/mmce.21105.
P. Kumar, T. Ali, M. M. M. Pai, “Electromagnetic metamaterials: A new paradigm of antenna design,” IEEE Access, vol. 9, pp. 18722–18751, 2021, doi: https://doi.org/10.1109/ACCESS.2021.3053100.
M. Alibakhshikenari et al., “A comprehensive survey of ‘Metamaterial transmission-line based antennas: Design, challenges, and applications,’” IEEE Access, vol. 8, pp. 144778–144808, 2020, doi: https://doi.org/10.1109/ACCESS.2020.3013698.
F. Ez-Zaki, H. Belahrach, A. Ghammaz, “Broadband microstrip antennas with Cantor set fractal slots for vehicular communications,” Int. J. Microw. Wirel. Technol., vol. 13, no. 3, pp. 295–308, 2021, doi: https://doi.org/10.1017/S1759078720000719.
A. Alemaryeen, S. Noghanian, “On-body low-profile textile antenna with artificial magnetic conductor,” IEEE Trans. Antennas Propag., vol. 67, no. 6, pp. 3649–3656, 2019, doi: https://doi.org/10.1109/TAP.2019.2902632.
M. N. Ramli, P. J. Soh, M. F. Jamlos, H. Lago, N. M. Aziz, A. A. Al-Hadi, “Dual-band wearable fluidic antenna with metasurface embedded in a PDMS substrate,” Appl. Phys. A, vol. 123, no. 2, p. 149, 2017, doi: https://doi.org/10.1007/s00339-017-0754-3.
S. Yan, P. J. Soh, G. A. E. Vandenbosch, “Low-profile dual-band textile antenna with artificial magnetic conductor plane,” IEEE Trans. Antennas Propag., vol. 62, no. 12, pp. 6487–6490, 2014, doi: https://doi.org/10.1109/TAP.2014.2359194.
T. Ijiguchi et al., “Circularly polarized one-sided directional slot antenna with reflector metal for 5.8-GHz DSRC operations,” IEEE Antennas Wirel. Propag. Lett., vol. 13, pp. 778–781, 2014, doi: https://doi.org/10.1109/LAWP.2014.2314962.
N. Rasool, H. Kama, M. Abdul Basit, M. Abdullah, “A low profile high gain ultra lightweight circularly polarized annular ring slot antenna for airborne and airship applications,” IEEE Access, vol. 7, pp. 155048–155056, 2019, doi: https://doi.org/10.1109/ACCESS.2019.2949683.
A. Liu, Y. Lu, L. Huang, “Low‐profile patch antennas with enhanced horizontal omnidirectional gain for DSRC applications,” IET Microwaves, Antennas Propag., vol. 12, no. 2, pp. 246–253, 2018, doi: https://doi.org/10.1049/iet-map.2017.0845.
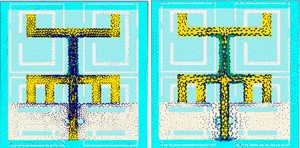