Protection of coherent pulse radars against combined interferences. 3. ALF-based digital adaptive system of sequential protection of programmed surveillance radars against combined interference
DOI:
https://doi.org/10.3103/S0735272722020029Abstract
This is the third paper of a series of articles devoted to modern methods of protection of coherent pulse radars against the combined interference (an additive mixture of internal noise, masking noise jamming and clutter). For a programmed surveillance radar, a prototype of the digital adaptive system for sequential space-time processing (STSP) of signals against the background of masking combined interference is described. This system is based on the 15-input 13-stage parallel adaptive lattice filter (ALF) and 5-stage sequential ALF that are built on a field programmable gate array (FPGA) and digital signal processor (DSP). Results of the prototype testing of adaptive STSP system using an additive mixture of simulated active noise jamming from five sources and digital recordings of real clutter in an operating radar are presented. These results have confirmed the high adaptation efficiency of the system based on adaptive lattice filters to the masking combined interference.
References
D. I. Lekhovytskiy, V. P. Riabukha, A. V. Semeniaka, D. V. Atamanskiy, Y. A. Katiushyn, “Protection of coherent pulse radars against combined interferences. 1. Modifications of STSP systems and their ultimate performance capabilities,” Radioelectron. Commun. Syst., vol. 62, no. 7, pp. 311–341, 2019, doi: https://doi.org/10.3103/S073527271907001X.
V. P. Riabukha, A. V. Semeniaka, Y. A. Katiushyn, D. V. Atamanskiy, “Protection of coherent pulse radars against combined interferences. 2. Analysis of influence of decorrelating factors on efficiency of adaptive sequential STSP,” Radioelectron. Commun. Syst., vol. 64, no. 11, pp. 573–583, 2021, doi: https://doi.org/10.3103/S0735272721110017.
Y. D. Shirman, S. T. Bagdasaryan, A. S. Malyarenko, D. I. Lekhovitskii, Radio Electronic Systems. Principles of Construction and Theory. Reference Book, [in Russian]. Moscow: Radiotekhnika, 2007.
J. Ward, “Space-time adaptive processing for airborne radar: Technical Report No. 1015,” Massachusetts, 1994. uri: https://apps.dtic.mil/sti/pdfs/ADA293032.pdf.
R. Klemm, Principles of Space-Time Adaptive Processing. Institution of Engineering and Technology, 2006, doi: https://doi.org/10.1049/PBRA021E.
W.-D. Wirth, Radar Techniques Using Array Antennas. Institution of Engineering and Technology, 2013, doi: https://doi.org/10.1049/PBRA026E.
J. R. Guerci, Space-Time Adaptive Processing for Radar, 2nd ed. Artech House, 2014.
W. L. Melvin, “Space-Time Adaptive Processing for Radar,” in Academic Press Library in Signal Processing: Volume 2: Communications and Radar Signal Processing, 2014, pp. 595–665.
M. Riedl, L. C. Potter, “Knowledge-aided Bayesian space-time adaptive processing,” IEEE Trans. Aerosp. Electron. Syst., vol. 54, no. 4, pp. 1850–1861, 2018, doi: https://doi.org/10.1109/TAES.2018.2805141.
W. Feng, Y. Guo, Y. Zhang, J. Gong, “Airborne radar space time adaptive processing based on atomic norm minimization,” Signal Process., vol. 148, pp. 31–40, 2018, doi: https://doi.org/10.1016/j.sigpro.2018.02.008.
H. Yuan, H. Xu, K. Duan, W. Xie, Y. Wang, “Cross-spectral metric smoothing-based GIP for space-time adaptive processing,” IEEE Geosci. Remote Sens. Lett., vol. 16, no. 9, pp. 1388–1392, 2019, doi: https://doi.org/10.1109/LGRS.2019.2897400.
Z. Li, T. Wang, “ADMM-based low-complexity off-grid space-time adaptive processing methods,” IEEE Access, vol. 8, pp. 206646–206658, 2020, doi: https://doi.org/10.1109/ACCESS.2020.3037652.
L. Xie, Z. He, J. Tong, W. Zhang, “A recursive angle-Doppler channel selection method for reduced-dimension space-time adaptive processing,” IEEE Trans. Aerosp. Electron. Syst., vol. 56, no. 5, pp. 3985–4000, 2020, doi: https://doi.org/10.1109/TAES.2020.2983533.
Y. Su, T. Wang, F. Tao, Z. Li, “A grid-less total variation minimization-based space-time adaptive processing for airborne radar,” IEEE Access, vol. 8, pp. 29334–29343, 2020, doi: https://doi.org/10.1109/ACCESS.2020.2972366.
X. Wang, Z. Yang, J. Huang, “Robust space‐time adaptive processing for airborne radar with coprime arrays in presence of gain and phase errors,” IET Radar, Sonar Navig., vol. 15, no. 1, pp. 75–84, 2021, doi: https://doi.org/10.1049/rsn2.12015.
H. Xiao, T. Wang, S. Zhang, C. Wen, “A robust refined training sample reweighting space–time adaptive processing method for airborne radar in heterogeneous environment,” IET Radar, Sonar Navig., vol. 15, no. 3, pp. 310–322, 2021, doi: https://doi.org/10.1049/rsn2.12034.
C. Vijaykumar Mahamuni, “Space-time adaptive processing techniques (STAP) for mitigation of jammer interference and clutter suppression in airborne radar systems: A MATLAB implementation-based study,” in SSRN Electronic Journal, 2020, pp. 1–6, doi: https://doi.org/10.2139/SSRN.3697565.
B. Tang, J. Tuck, P. Stoica, “Polyphase waveform design for MIMO radar space time adaptive processing,” IEEE Trans. Signal Process., vol. 68, pp. 2143–2154, 2020, doi: https://doi.org/10.1109/TSP.2020.2983833.
D. M. Piza, S. N. Romanenko, D. S. Semenov, “Increase of efficiency of compensation of the active component of combined interference,” Radio Electron. Comput. Sci. Control, no. 3, pp. 7–14, 2019, doi: https://doi.org/10.15588/1607-3274-2019-3-1.
D. M. Piza, D. S. Semenov, S. V. Morshchavka, “Efficiency estimation of discrete algorithms for adaptation of weight coefficients in space-time processing of radar signals,” Radioelectron. Commun. Syst., vol. 62, no. 1, pp. 6–11, 2019, doi: https://doi.org/10.3103/S0735272719010023.
D. M. Piza, S. N. Romanenko, D. S. Semenov, “Enhancing efficiency of space-time processing of radar signals under exposure of combined interferences,” Radioelectron. Commun. Syst., vol. 63, no. 5, pp. 257–264, 2020, doi: https://doi.org/10.3103/S0735272720050040.
V. P. Ryabukha, D. S. Rachkov, A. V. Semeniaka, I. A. Katiushyn, “Estimation of spatial weight vector fixation interval for sequential space-time signal processing against the background of combined interferences,” Radioelectron. Commun. Syst., vol. 55, no. 10, pp. 443–451, 2012, doi: https://doi.org/10.3103/S0735272712100020.
D. I. Lekhovytskiy, V. P. Riabukha, D. V. Atamanskiy, A. V. Semeniaka, D. S. Rachkov, “Lattice filtration theory. Part I: One-dimensional lattice filters,” Telecommun. Radio Eng., vol. 80, no. 5, pp. 41–79, 2021, doi: https://doi.org/10.1615/TelecomRadEng.2021039186.
“System of protection of main (information) channels from noise interference,” UA Patent No. 112834, 2016.
V. P. Riabukha, A. V. Semeniaka, Y. A. Katiushyn, D. V. Atamanskiy, “Selection of parameters for band-diagonal regularization of maximum likelihood estimates of Gaussian interference correlation matrices and their inverses,” Radioelectron. Commun. Syst., vol. 64, no. 5, pp. 229–237, 2021, doi: https://doi.org/10.3103/S0735272721050010.
V. P. Riabukha, A. V. Semeniaka, Y. A. Katiushyn, D. V. Atamanskiy, “Selection of number of compensatory channels and placement of receivers with non-identical frequency response at phased-array radar under Gaussian noise jamming,” Radioelectron. Commun. Syst., vol. 65, no. 1, pp. 1–10, 2022, doi: https://doi.org/10.3103/S0735272722010010.
N. Hasanikhah, S. Amin-Nejad, G. Darvish, M. R. Moniri, “Comparison of practical methods for an efficient FPGA implementation of STAP,” Int. J. Electron., vol. 106, no. 9, pp. 1320–1331, 2019, doi: https://doi.org/10.1080/00207217.2019.1590863.
D. I. Lekhovytskiy, A. V. Semeniaka, V. P. Riabukha, D. S. Rachkov, “Recursive algorithms of adaptive lattice filters adjustment,” Technol. Des. Electron. Equip., no. 2–3, pp. 26–32, 2016, doi: https://doi.org/10.15222/TKEA2016.2-3.26.
D. I. Lekhovytskiy, “Adaptive lattice filters for systems of space-time processing of non-stationary Gaussian processes,” Radioelectron. Commun. Syst., vol. 61, no. 11, pp. 477–514, 2018, doi: https://doi.org/10.3103/S0735272718110018.
V. P. Riabukha, A. V. Semeniaka, Y. A. Katiushyn, “Mathematical models of cross-correlated and uncorrelated Gaussian noise jamming from external sources,” Radioelectron. Commun. Syst., vol. 64, no. 3, pp. 147–154, 2021, doi: https://doi.org/10.3103/S0735272721030043.
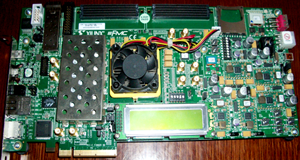