Tunable reflective structures based on weak ferromagnetics and their application as tunable sub-terahertz resonators
DOI:
https://doi.org/10.3103/S0735272719080016Keywords:
weak ferromagnetic, iron borate, hematite, Fabry–Perot resonator, metasurface, sub-terahertz rangeAbstract
Electromagnetic eigenwaves in a weak ferromagnetic with anisotropy of the “easy plane” under magnetization in this plane are investigated. It is shown that at frequencies of anti-resonances the weak ferromagnetic can be considered as environment with characteristic impedance close to zero. The polarization of eigenwaves in such environment is analyzed on the example of high-temperature weak ferromagnetics—hematite and iron borate. It is proposed to consider the weak ferromagnet–metal structure as an element of the metasurface with a controlled phase shift of the reflected wave. A tunable Fabry–Perot resonator with a weak ferromagnet–metal structure (ferromagnet is of sub-wave thickness) is considered and the corresponding frequency-field dependencies in the sub-terahertz range are studied. It is shown that such resonator combines rather high Q-factor with a possibility of magnetic tuning of its resonance frequency. The maximum local slope of the field dependence of the resonator frequency reaches 0.3 MHz/Oe using a hematite layer and 0.6 MHz/Oe using an iron borate layer of 10 mm thickness. The resonator unloaded quality factor for the main mode varies within 300–1400 and 250–1000, respectively.References
LIBERAL, Inigo; ENGHETA, Nader. “Near-zero refractive index photonics,” Nature Photonics, v.11, p.149, 2017. DOI: https://doi.org/10.1038/nphoton.2017.13.
LIBERAL, Inigo; ENGHETA, Nader. “The rise of near-zero-index technologies,” Science, v.358, n.6370, p.1540, 2017. DOI: http://doi.org/10.1126/science.aaq0459.
MARCOS, Joao S.; SILVEIRINHA, Mario G.; ENGHETA, Nader. “m-near-zero supercoupling,” Phys. Rev. B, v.91, 2015. DOI: https://doi.org/10.1103/physrevb.91.195112.
DU, B.; XU, Z.; WANG, J.; XIA, S. “Magnetically tunable ferrite-dielectric left-handed metamaterial,” PIER C, v.66, p.21, 2016. DOI: https://doi.org/10.2528/pierc16042806.
LI, Wei; WEI, Jia; WANG, Wei; HU, Dawei; LI, Yukin; GUAN, Jianguo. “Ferrite-based metamaterial microwave absorber with absorption frequency magnetically tunable in a wide range,” Materials Design, v.110, p.27, 2016. DOI: https://doi.org/10.1016/j.matdes.2016.07.118.
WANG, Yongxing; QIN, Yue; SUN, Zhouzhou; XU, Ping. “Magnetically controlled zero-index metamaterials based on ferrite at microwave frequencies,” J. Phys. D: Appl. Phys., v.49, p.45106, 2016. DOI: https://doi.org/10.1088/0022-3727/49/40/405106.
LU, Conghui; RONG, Cancan; HUANG, Xiutao; HU, Zhaoyang; TAO, Xiong; WANG, Shengming; CHEN, Junfeng; LIU, Minghai. “Investigation of negative and near-zero permeability metamaterials for increased efficiency and reduced electromagnetic field leakage in a wireless power transfer system,” IEEE Trans. Electromagnetic Compatibility, p.1, 2018. DOI: https://doi.org/10.1109/temc.2018.2865520.
SILVEIRINHA, Mario; ENGHETA, Nader. “Tunneling of electromagnetic energy through subwavelength channels and bends using ε-near-zero materials,” Phys. Rev. Lett., v.97, p.157403, 2006. DOI: https://doi.org/10.1103/physrevlett.97.157403.
LIBERAL, I.; MAHMOUD, A.M.; ENGHETA, N. “Geometry-invariant resonant cavities,” Nat. Commun., v.7, p.10989, 2016. DOI: https://doi.org/10.1038/ncomms10989.
SILVEIRINHA, Mario G. “Trapping light in open plasmonic nanostructures,” Phys. Rev. A, v.89, p.023813, 2014. DOI: https://doi.org/10.1103/PhysRevA.89.023813.
KAGANOV, M.I.; PUSTYL’NIK, N.B.; SHALAEVA, T.I. “Magnons, magnetic polaritons, magnetostatic waves,” Phys. Usp., v.40, p.181, 1997. DOI: https://doi.org/10.1070/PU1997v040n02ABEH000194.
PIMENOV, Yu.V. Linear Macroscopic Electrodynamics [in Russian]. Intellect, 2008.
BUCHEL’NIKOV, V.D.; BABUSHKIN, A.V.; BYCHKOV, I.V. “Electromagnetic-wave reflectivity of the surface of a cubic-ferrite plate,” Phys. Solid State, v.45, n.4, p.696, 2003. DOI: https://doi.org/10.1134/1.1569009.
BUTKO, L.N.; BUCHELNIKOV, V.D.; BYCHKOV, I.V. “Absorption coefficient of electromagnetic waves in a layered structure ‘non-magnetic conductor-ferrite’,” Bull. Chelyabinsk State Univ., n.24, p.50, 2010. URI: https://elibrary.ru/item.asp?id=15279626.
ZAVISLYAK, I.V.; CHUMAK, H.L. “Controlled reflective surfaces based on ferrite layer,” Radiofiz. Electron., n.1, p.3, 2019. DOI: https://doi.org/10.15407/rej2019.01.003.
IVANOV, B.A. “Spin dynamics of antiferromagnets under femtosecond laser pulses (review),” Low Temperature Phys., v.40, n.2, p.119, 2014. URI: http://dspace.nbuv.gov.ua/handle/123456789/119411.
DZYALOSHINSKY, I. “A thermodynamic theory of ‘weak’ ferromagnetism of antiferromagnetics,” J. Phys. Chem. Solids, v.4, n.4, p.241, 1958. DOI: https://doi.org/10.1016/0022-3697(58)90076-3.
MORIYA, Toru. “Anisotropic superexchange interaction and weak ferromagnetism,” Phys. Rev., v.120, n.1, 1960. DOI: https://doi.org/10.1103/physrev.120.91.
BOLOTIN, D.D.; MACKSIMOVA, E.M.; STRUGATSKY, M.B. “Comparative analysis of crystalomagnetic structure of iron borate and hematite,” Scientific Notes of Taurida National V.I. Vernadsky University. Series: Physics and Mathematics Sciences, v.23, n.3, p.149, 2010. URI: http://jphystech.cfuv.ru/wp-content/uploads/2017/05/015_bolotin.pdf.
TUROV, E.A.; ET. AL. Symmetry and Physical Properties of Antiferromagnets [in Russian]. Moscow: Fizmatlit, 2001.
POPOV, M.A.; ZAVISLYAK, I.V.; CHUMAK, H.L.; STRUGATSKY, M.B.; YAGUPOV, S.V.; SRINIVASAN, G. “Ferromagnetic resonance in a single crystal of iron borate and magnetic field tuning of hybrid oscillations in a composite structure with a dielectric: Experiment and theory,” J. Appl. Phys., v.118, p.013903, 2015. DOI: https://doi.org/10.1063/1.4923413.
AVAEVA, I.G.; KRAVCHENKO, V.B.; LISOVSKY, F.V.; SOBOLEV, A.T.; SHAPOVALOV, V.I. “Preparation and study of single crystals of the antiferromagnet FeBO3,” in: Physical and Physico-Chemical Properties of Ferrites [in Russian]. Minsk: Nauka i Tekhnika, 1975, p.157-163.
VELIKOV, L.V.; RUDASHEVSKII, E.G. “Antiferromagnetic resonance in hematite in the weakly ferromagnetic state,” Soviet Phys. JETP, v.29, n.5, p.836, 1969. URI: http://www.jetp.ac.ru/cgi-bin/r/index/r/56/5/p1557?a=list.
KOCHARIAN, K.N.; AVAKYAN, A.A.; PRPNIAN, V.G.; SARGSYAN, E.L.; SELEZNEV, V.N.; MALNEV, V.V.; YAGUPOV, S.V. “Submillimeter electrodynamics of iron borate,” FTT, v.36, n.3, p.839, 1994. URI: http://journals.ioffe.ru/articles/16424.
TIKHONOV, V.V.; BOYARSKY, D.A.; POLYAKOVA, O.N.; DZARDANOV, A.L.; GOLTSMAN, G.N. “Laboratory studies of the radiophysical and dielectric properties of minerals and rocks in the microwave range,” Space Research Institute RAS, 2011.
TARASENKO, V.V.; KHARITONOV, V.D. “Surface magnetostatic waves in uniaxial antiferromagnets,” JETP, v.33, n.6, p.2321, 1971. URI: http://www.jetp.ac.ru/cgi-bin/r/index/r/60/6/p2321?a=list.
GUREVICH, A.G. Magnetic Resonance in Ferrites and Antiferromagnets [in Russian]. Moscow: Nauka, 1973.
PANKRATZ, A.I.; PETRAKOVSKIY, G.A.; SMYK, A.F. “Dispersion rule of magnetostatic oscillations and magnetodynamic resonance in a plate of a weak ferromagnet FeBO3,” DAN USSR, v.294, n.5, p.1097, 1987. URI: https://elibrary.ru/item.asp?id=24104949.
GRIGORIEV, A.D. Electrodynamics and Microwave Technology [in Russian]. Moscow: Vyssh. Shkola, 1990.
REMNEV, M.A.; KLIMOV, V.V. “Metasurfaces: a new look at Maxwell’s equations and new ways to control light,” Phys. Usp., v.61, n.2, p.157, 2018. DOI: https://doi.org/10.3367/UFNe.2017.08.038192.
ANDRADE-NETO, A.V. “Dielectric function for free electron gas: comparison between Drude and Linhard models,” Revista Brasileira de Ensino de Fisica, v.39, n.2, 2017. DOI: https://doi.org/10.1590/1806-9126-rbef-2016-0206.
MIKAEYAN, A.L. Optical Methods in Computer Science [in Russian]. Moscow: Nauka, 1990.
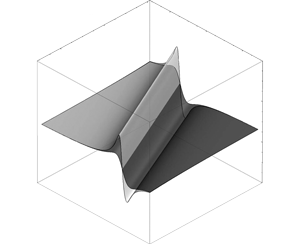